A Framework for Development of Quality Control Model for Indian Ready Mixed Concrete Industry
Quality Control of Ready Mixed Concrete can be divided into three convenient areas like forward control, immediate control and retrospective control. SQC application proves to be a vital tool which can be used effectively for quality and productivity improvement for infrastructure projects. Statistical Quality Control can be effectively applied to RMC industry for online (during production) and also offline (before and after production) quality monitoring and control. SQC and particularly SPC techniques have potentiality to improve efficiency and profitability of the organization. However, in absence of proper and effective quality monitoring systems in most of the batching plants in India, they are lagging behind from their western counterparts in terms of operational procedures, product quality and economy in manufacture of concrete. Hence there is a need to analyze the applicability of scientific quality monitoring techniques to RMC in India. The proposed Quality Control Model for Indian RMC industry is a SQC based, simplified user–friendly model, which if adopted, we think, would make Indian RMC industry much more organized and efficient in terms of production and operation.
The most interesting aspect of RMC industry is its remarkable growth. In United States, in 1925, there were about twenty RMC operators, by 1929 this number had grown to hundred and today there are more than ten thousand operators. In United Kingdom between 1950 and 1974, about thirty one million cubic meters of RMC was produced at its peak. Today this production have exceeded ninety one million cubic meters. Since its inception, major developmental research work pertaining to production and operational procedures has been carried out in UK.
From this growth pattern, it is obvious that the RMC plants had something to offer. The consumer wanted his concrete delivered to the job in a ready-to-place condition. This growth has been accompanied by improvements in the equipment and methods used by the ready mixed operator. Volume batching has completely been replaced by weigh batching and presently computerized weigh batchers are used in most of the batching plants. Aggregates are stored in properly installed bins and cement and flyash are stored in silos. Conveyors are used to transport the aggregates. Cement and flyash is pumped into the central mixer with pneumatic pumps. Electronic moisture meters, digital admixture dispensers are used in fully automatic batching plants. This introduction of improved batching equipment has made possible the exact and scientific formulation of concrete mix. Concrete designed to meet the specific requirements can now be ordered directly from the ready mixed operators.
Quality Control of RMC can be divided into three convenient areas like forward control, immediate control and retrospective control (Dewar and Anderson, 1988). Forward control basically deals with procedures of quality control to be followed before the production process. This covers (i) materials storage, (ii) monitoring of quality of materials, (iii) modification of mix design, (iv) plant maintenance, (v) calibration of equipment and (vi) plant and transit mixer condition. Immediate control is concerned with instant action to control the quality of concrete during production or that of deliveries closely following production. This covers (i) weighing – correct reading of batch data and accurate weighing, (ii) visual observation and testing of concrete during production and delivery (assessment of uniformity, cohesion, workability, adjustment of water content) and (iii) making corresponding adjustments at the plant automatically or manually to batched quantities to allow for observed, measured or reported changes in materials or concrete qualities. Retrospective control primarily deals with the quality control procedures after production. This covers (i) sampling of concrete, testing and monitoring of results, (ii) weighbridge checks of laden and unladen vehicle weights, (iii) Stock control of materials and (iv) diagnosis and correction of identified faults.
In India RMC was launched about two decades ago. Lack of proper manuals, higher initial costs than conventional site mixed concrete, high initial investments for installation of automatic batching plants and also lack of awareness were the major causes that led to an initial setback to the RMC industry. In the present era rapid urbanization has resulted in increase in demand for multistoried housing complexes, commercial complexes like shopping malls, retail units, multiplexes, multistoried office buildings and other real estate projects have largely increased the demand of good quality concrete to make the structures adequately safe and durable. Use of good quality concrete is also one of the basic requirements to make the structure earthquake resistant. Problems in availability of land in urban cities particularly the metro and mega cities like Delhi, Mumbai, Chennai, Kolkata, Ahmedabad, Bangalore, Pune etc. have increased the need for vertical expansion due to restrictions and constraints in horizontal expansion. Thereby construction of tall structures has become an essential requirement of urban cities. Thus in course of time awareness of the advantages of using RMC and realization of the fact that the conventional concrete may result in higher lifecycle cost due to higher maintenance costs made the construction industry to adopt RMC as a better option economically and qualitatively. But still in India about 2% of the total cement produced is utilized in RMC production against 70% of the cement produced being utilized in RMC in UK and US.
In this paper, an attempt has been made to develop a framework for Statistical Quality Control (SQC) based quality control and monitoring model for Indian RMC industry.
To develop the SQC based model for quality control and monitoring for Indian RMC industry data have been collected from four operational RMC plants, two of which are from Ahmedabad and two from Delhi. One of the plant from Ahmedabad is fully automatic and has a production capacity of 30 cum / hr. This is referred as Case 1. The silo for this plant for storing cement is of 100 MT capacity and the silo for storing flyash is of 80 MT capacity. Cement and flyash is pumped with pneumatic pumps. The typical grades of concrete produced are M15 to M50 with both 43 and 53 grade cements. The other plant from Ahmedabad is semi automatic with a production capacity of 15 cum/hr. This plant has been set up by a contracting organization for supplying concrete for their in-house projects. This is referred as CASE 2. Here silos are not available for storage of cement / flyash and raw materials are transported into the central mixer through screw conveyors. The grades of concrete produced are M20 and M25 with 43 and 53 grade of cement. The plants of Delhi are fully automatic and have production capacity of 60 cum / hr. Cement and flyash are stored in silos and conveyors are available for transporting raw materials. The concrete produced are M15 to M50 with 43 and 53 grade cement. High strength concrete upto 80 N/mm2(Mpa) can also be produced. These two plants are referred as Case 3 and Case 4 respectively.
Data pertaining to total quality management system for production and operation of RMC like cube compressive strength of 7 days and 28 days concrete grades of M15, M20, M25, M30, M40, tests of raw materials like cement, coarse aggregates, fine aggregates, admixtures, water, details of present equipment used, cycle time for production, production capacity of the respective plants under study, methods for transportation of raw materials, transportation methods for produced concrete, details of concrete mix designs have been collected. Also observations pertaining to the lacunae in the present production, monitoring and operational procedures and the present day to day problems faced by RMC industry have been noted.
A comparative presentation of all the samples collected from Cases 1, 2, 3, and 4 along with the calculated mean, plant standard deviation, target mean strength, target mean range and coefficient of variation are presented in table 2. As per Dewar and Anderson, (1988), Target Mean Strength (TMS) = fck + 2 σ…. (1) where fck is the characteristic cube compressive strength at 28 days, σ = plant standard deviation. Target Mean Range (TMR) = 1.128 x assumed standard deviation (plant standard deviation)….. (2).
Table 2 has been formulated using Excel wherein the mean strength of the data of each slot for a specified concrete grade is calculated and presented in column (6). Column (5) represents the values of plant standard deviation as obtained from the calculation of the values of the compressive strengths of the collected samples of different batches of the same slot and mix design. It is more logical to use the values of the plant standard deviations for analysis for target mean strengths greater than 27 N/mm2 (Dewar and Anderson, 1988). From the calculated results it is observed that for four case studies under consideration the value of standard deviation obtained in the plant varies from values as low as 1.92 to as high as 6.57. This reflects the fact that a different degree of quality control is maintained by each plant. The value of standard deviation of 1.92 is for a plant which is semi-automatic. Since this plant is not a commercial RMC plant and is set up by a contracting organization to supply concrete for construction of a management institute, location and working conditions of the plant are excellent and most of operating conditions are under control and the quantity of concrete produced, is of range of 600 to 900 cum per month. Due to this limited production they might be able to maintain good degree of quality control, thus having lower standard deviation values. On contrary for a plant as in case 3 located in Delhi which is fully automatic and has latest imported equipment, but is not able to maintain a good degree of quality control, which is reflected from a very high value of standard deviation like 6.57 may be due to the pressure of producing and delivering very high quantity of concrete which may be of the range of 500 to 800 cum per day during peak demands. Thus the present work aims in developing an effective quality monitoring and control model which will definitely benefit the commercial batching plants located in metros and major cities of India which have a target of producing superior quality concrete of quantities as high as 1000 to 1200 cum per day.
Target Mean Strength (TMS) for each mix is calculated as per equation (1) and the Target Mean Range (TMR) as per the relationship TMR = 1.128 σ (Dewar and Anderson, 1988). The CUSUM for range aims in monitoring the difference between two consecutive values of compressive strength obtained and also monitors the difference between highest and lowest value of the strength obtained in a specified grade of concrete of different batches of the same slot produced from same mix design. The coefficient of variation presented in column (9) is a good indicator of the degree of quality control maintained by the plant. As per the results obtained the coefficient of variation varies from 0.06 (Case 2) to 0.164 (Case 1). The average coefficient of variation of Case 1 is 0.118, Case 2 is 0.068, Case 3 is 0.131 and of CASE 4 is 0.116.
These results reflect the fact that amongst the four cases under analysis best quality control is maintained by the plant of Case 2, followed by Case 4, Case 1 and Case 3.
Testing of Raw Materials, Fresh Concrete and Hardened Concrete
The incoming materials particularly raw materials like cement, coarse aggregate 10mm, coarse aggregate 20mm, fine aggregate, admixtures should be subjected to testing as per adopted acceptance sampling plan. A proposed scheme for the basic tests to be conducted for raw materials, fresh concrete and hardened concrete along with their frequency of testing is given in Table 3.

A standard QA /QC laboratory equipped with latest testing equipments should be established at site. Minimum two lab technicians should support two QA /QC engineers. The QA / QC engineers should report to QA / QC Incharge who in turn should report to QA/QC Manager. The incoming raw materials (cement, fine aggregates, coarse aggregates 10mm, coarse aggregates 20mm, flyash, admixtures etc.) should be tested with visual checks as per the testing procedures laid down by the respective QA/QC department of the plant. The raw materials found unsatisfactory in the visual checks should be rejected and as per requirement confirmatory tests should be conducted before rejection. The supplier should be immediately informed about the rejection of his material and should be warned so that if he repeats the same, his contract would be terminated. Standard tests for raw materials, fresh concrete and hardened concrete have to be performed as per Indian Standard (IS) testing procedures. The details of these tests along with the respective IS codes and frequency of testing is given in table
The materials qualifying in the respective tests are accepted and aggregates are stored in respective aggregate storage bins. Cement and flyash should be stored in cement silo and flyash silo respectively. It is desirable to transport the cement in bulkers and directly pump it into the silo through pneumatic pumps. The cement if required to be stored in godowns should be well protected from rains. The godown should be covered on all sides and should be provided with adequate size of lockable entry or exit gates. Cement should always be stored on a plain cement concrete base which is about 200 mm thick or brickwork base which is about 300 mm above the ground. The oldest lot should be used first. It is always better to cover the cement bags with polythene sheets particularly in pre-monsoon and monsoon seasons. The workforce involved in handling cement bags or bulk cement should compulsorily be provided with personal protective equipments like safety helmets, safety shoes hand gloves and safety goggles. During pumping of cement into the silos the workmen should take utmost care so that the cement due to back hammering effect does not create major accidents like severe eye injuries. The workmen should compulsorily wear safety goggles and helmet while working with the pneumatic pumps.
The stored raw materials should be weighed (as per the weights of design mix) in the respective weigh hoppers fitted with load cells. These weighed materials through screw conveyors or loaders should be transported to the central mixer. Measured quantity of water through water meter and admixtures through admixture dispensers should be added into the central mixer after dry mixing of cement, flyash, coarse aggregates and fine aggregates. After addition of water and admixtures the final mixing should be carried out for about 30 seconds. The entire loading process takes about 90 seconds. Thus the ideal cycle time for each of concrete is about 120 seconds. The capacity of each batch may be of 0.5 m3, 1 m3 and 2 m3 depending upon the capacity of the plant.
The RMC thus produced have to undergo tests of fresh concrete like slump tests and corresponding temperatures of the concrete should be noted. If the concrete is produced in summers when the outside temperature is about 40 to 45 degree Celsius the drum of the transit mixer should be covered with wet jute cloth and desirably some ice cubes should be added to the fresh concrete. The slump of the mixes to be placed by concrete pumps should be at least 180mm to 200 mm at the time of pumping. Thus use of flyash in the mix is always desirable for pumpable mixes as flyash due to ball bearing action increases the pumpability of a mix. The slump of concrete to be placed by crane and concrete bucket or by elevator hoists should be about 100 to 120mm at site. Minimum 6 cubes (150mm x 150mm x 150mm) should be prepared for each batch of concrete produced as per standard procedures. The cubes should always be casted in a cool shady place and in no case should the casting procedure be carried out under hot sun. The cubes should be air cooled for about 24 hours and then placed into the curing tank for curing. The curing tank should be completely filled with water and desirably should contain different compartments for 3 days, 7 days and 28 days testing. The fresh cubes should be marked with the specified grade of concrete, date of casting and should be placed in a jute gunny bag and after tying the mouth of the bag and putting a plastic tag of the grade and date of casting of the concrete, the bag should be placed in the desired compartment of the curing tank. Generally if the requirement is that the concrete needs to be tested for 7 days and 28 days compressive strength, then 3 cubes should be packed in the jute gunny bag and placed into the compartment for 7 days curing and other 3 cubes should be placed in the compartment for 28 days curing. As per client requirement if 3 days strength also needs to be tested then total 9 cubes should be casted. Following a systematic procedure of casting and curing the cubes improves the degree of quality control of the plant. The cubes should be tested in hydraulically operated compressive strength testing machine and desirably the manually lever operated strength testing equipment should be avoided. Care should be taken to ensure that the cube is uniformly seated before application of the load and the rate of loading should be uniform and desirably 140 kg /cm2/min. The failure load of the three cubes should be noted separately and the corresponding compressive strength should be calculated. The average of three cube strengths can be considered as the observed strength of a sample.
The fresh concrete thus prepared is loaded in transit mixers and transported to respective sites. The time from batching to placing of the concrete at the site should not exceed 2 hours. If the concrete is transported in summers the drum of the transit mixer should be covered with wet jute cloth and during extremely high temperatures ice cubes should added in the concrete. No additional water should be added in the concrete enroute. Before placing, the concrete should be tested for desirable slump, temperature, uniformity and cohesiveness and if found ok should be placed at the respective site.
The 7 days and 28 days strength obtained should be monitored using CUSUM and V-mask. This technique should be used as a daily monitoring tool and when the results of about four to five samples are obtained the data should be plotted using Excel / SPSS software where the x- axis of the graphical representation of the CUSUM plot (mean strength, range and correlation) represents sample number and the corresponding y- axis represents the CUSUM value in N / mm2 or Mpa. The V-mask plotted in a transparent paper should be superimposed on the obtained CUSUM plot by placing the lead point of the mask on each and every obtained CUSUM value starting from the first sample. If the plot remains within the boundary of the mask, the process is in control. If the plot crosses the boundary of the mask, a significant change has occurred in the process mean and an action is required. The root cause of the problem need to be investigated and accordingly action should be taken. A change in cement content can be calculated by the empirical relationship proposed by Dewar and Anderson (1988), and trial cubes can be casted and tested with the modified mix design value. The proposed Quality Control Model for Indian RMC Industry is presented in Figure 1.
Introduction
As per Indian Standard code of practice (IS 4926) Ready Mixed Concrete (RMC) is defined as the concrete delivered in plastic condition and requiring no further treatment before being placed in position in which it is to set and harden. Instead of being batched and mixed on site, concrete is delivered for placing from central batching plant. History reveals that RMC was patented in Germany in 1903, but the means of transporting it had not developed sufficiently well to enable the concept to be exploited. There were significant developments in USA in the first quarter of 20thcentury. The first delivery of RMC was made in Baltimore in 1913, and the transitmixer was born in 1926. In 1931 K.O. Ammentorp, erected a plant at Bedfont, west of London and launched a company named as Ready Mixed Concrete Ltd. At the same time companies like British Steel Piling Company, Scientific Concrete Co. Ltd, Jaeger System Ltd, became interested and entered into RMC business.The most interesting aspect of RMC industry is its remarkable growth. In United States, in 1925, there were about twenty RMC operators, by 1929 this number had grown to hundred and today there are more than ten thousand operators. In United Kingdom between 1950 and 1974, about thirty one million cubic meters of RMC was produced at its peak. Today this production have exceeded ninety one million cubic meters. Since its inception, major developmental research work pertaining to production and operational procedures has been carried out in UK.
From this growth pattern, it is obvious that the RMC plants had something to offer. The consumer wanted his concrete delivered to the job in a ready-to-place condition. This growth has been accompanied by improvements in the equipment and methods used by the ready mixed operator. Volume batching has completely been replaced by weigh batching and presently computerized weigh batchers are used in most of the batching plants. Aggregates are stored in properly installed bins and cement and flyash are stored in silos. Conveyors are used to transport the aggregates. Cement and flyash is pumped into the central mixer with pneumatic pumps. Electronic moisture meters, digital admixture dispensers are used in fully automatic batching plants. This introduction of improved batching equipment has made possible the exact and scientific formulation of concrete mix. Concrete designed to meet the specific requirements can now be ordered directly from the ready mixed operators.
Quality Control of RMC can be divided into three convenient areas like forward control, immediate control and retrospective control (Dewar and Anderson, 1988). Forward control basically deals with procedures of quality control to be followed before the production process. This covers (i) materials storage, (ii) monitoring of quality of materials, (iii) modification of mix design, (iv) plant maintenance, (v) calibration of equipment and (vi) plant and transit mixer condition. Immediate control is concerned with instant action to control the quality of concrete during production or that of deliveries closely following production. This covers (i) weighing – correct reading of batch data and accurate weighing, (ii) visual observation and testing of concrete during production and delivery (assessment of uniformity, cohesion, workability, adjustment of water content) and (iii) making corresponding adjustments at the plant automatically or manually to batched quantities to allow for observed, measured or reported changes in materials or concrete qualities. Retrospective control primarily deals with the quality control procedures after production. This covers (i) sampling of concrete, testing and monitoring of results, (ii) weighbridge checks of laden and unladen vehicle weights, (iii) Stock control of materials and (iv) diagnosis and correction of identified faults.
In India RMC was launched about two decades ago. Lack of proper manuals, higher initial costs than conventional site mixed concrete, high initial investments for installation of automatic batching plants and also lack of awareness were the major causes that led to an initial setback to the RMC industry. In the present era rapid urbanization has resulted in increase in demand for multistoried housing complexes, commercial complexes like shopping malls, retail units, multiplexes, multistoried office buildings and other real estate projects have largely increased the demand of good quality concrete to make the structures adequately safe and durable. Use of good quality concrete is also one of the basic requirements to make the structure earthquake resistant. Problems in availability of land in urban cities particularly the metro and mega cities like Delhi, Mumbai, Chennai, Kolkata, Ahmedabad, Bangalore, Pune etc. have increased the need for vertical expansion due to restrictions and constraints in horizontal expansion. Thereby construction of tall structures has become an essential requirement of urban cities. Thus in course of time awareness of the advantages of using RMC and realization of the fact that the conventional concrete may result in higher lifecycle cost due to higher maintenance costs made the construction industry to adopt RMC as a better option economically and qualitatively. But still in India about 2% of the total cement produced is utilized in RMC production against 70% of the cement produced being utilized in RMC in UK and US.
In this paper, an attempt has been made to develop a framework for Statistical Quality Control (SQC) based quality control and monitoring model for Indian RMC industry.
Case Study
Mode of Data Collection
To develop the SQC based model for quality control and monitoring for Indian RMC industry data have been collected from four operational RMC plants, two of which are from Ahmedabad and two from Delhi. One of the plant from Ahmedabad is fully automatic and has a production capacity of 30 cum / hr. This is referred as Case 1. The silo for this plant for storing cement is of 100 MT capacity and the silo for storing flyash is of 80 MT capacity. Cement and flyash is pumped with pneumatic pumps. The typical grades of concrete produced are M15 to M50 with both 43 and 53 grade cements. The other plant from Ahmedabad is semi automatic with a production capacity of 15 cum/hr. This plant has been set up by a contracting organization for supplying concrete for their in-house projects. This is referred as CASE 2. Here silos are not available for storage of cement / flyash and raw materials are transported into the central mixer through screw conveyors. The grades of concrete produced are M20 and M25 with 43 and 53 grade of cement. The plants of Delhi are fully automatic and have production capacity of 60 cum / hr. Cement and flyash are stored in silos and conveyors are available for transporting raw materials. The concrete produced are M15 to M50 with 43 and 53 grade cement. High strength concrete upto 80 N/mm2(Mpa) can also be produced. These two plants are referred as Case 3 and Case 4 respectively.
Data pertaining to total quality management system for production and operation of RMC like cube compressive strength of 7 days and 28 days concrete grades of M15, M20, M25, M30, M40, tests of raw materials like cement, coarse aggregates, fine aggregates, admixtures, water, details of present equipment used, cycle time for production, production capacity of the respective plants under study, methods for transportation of raw materials, transportation methods for produced concrete, details of concrete mix designs have been collected. Also observations pertaining to the lacunae in the present production, monitoring and operational procedures and the present day to day problems faced by RMC industry have been noted.
Classification of Mixes and Comparative Analysis of Case Studies
The various concrete mixes collected from Case 1, 2, 3 and 4 have been given a specific designation based on the grade of concrete, slot / batch and specific case from where the batch has been collected. These designations prove to be very useful in referring the concerned mixes during the analysis. For Mix “1 M20 C1,” “1”refers to the slot for which this mix designs is followed (20.05.06 to 04.06.06). “M20” is the specified grade of concrete where “M” is Mix and 20 is the characteristic compressive strength at 28 days which for this case is 20 N/mm2 or 20 Mpa. “C1” represents Case 1. Details of this designation are presented in Table1.
A comparative presentation of all the samples collected from Cases 1, 2, 3, and 4 along with the calculated mean, plant standard deviation, target mean strength, target mean range and coefficient of variation are presented in table 2. As per Dewar and Anderson, (1988), Target Mean Strength (TMS) = fck + 2 σ…. (1) where fck is the characteristic cube compressive strength at 28 days, σ = plant standard deviation. Target Mean Range (TMR) = 1.128 x assumed standard deviation (plant standard deviation)….. (2).

Table 2 has been formulated using Excel wherein the mean strength of the data of each slot for a specified concrete grade is calculated and presented in column (6). Column (5) represents the values of plant standard deviation as obtained from the calculation of the values of the compressive strengths of the collected samples of different batches of the same slot and mix design. It is more logical to use the values of the plant standard deviations for analysis for target mean strengths greater than 27 N/mm2 (Dewar and Anderson, 1988). From the calculated results it is observed that for four case studies under consideration the value of standard deviation obtained in the plant varies from values as low as 1.92 to as high as 6.57. This reflects the fact that a different degree of quality control is maintained by each plant. The value of standard deviation of 1.92 is for a plant which is semi-automatic. Since this plant is not a commercial RMC plant and is set up by a contracting organization to supply concrete for construction of a management institute, location and working conditions of the plant are excellent and most of operating conditions are under control and the quantity of concrete produced, is of range of 600 to 900 cum per month. Due to this limited production they might be able to maintain good degree of quality control, thus having lower standard deviation values. On contrary for a plant as in case 3 located in Delhi which is fully automatic and has latest imported equipment, but is not able to maintain a good degree of quality control, which is reflected from a very high value of standard deviation like 6.57 may be due to the pressure of producing and delivering very high quantity of concrete which may be of the range of 500 to 800 cum per day during peak demands. Thus the present work aims in developing an effective quality monitoring and control model which will definitely benefit the commercial batching plants located in metros and major cities of India which have a target of producing superior quality concrete of quantities as high as 1000 to 1200 cum per day.
Target Mean Strength (TMS) for each mix is calculated as per equation (1) and the Target Mean Range (TMR) as per the relationship TMR = 1.128 σ (Dewar and Anderson, 1988). The CUSUM for range aims in monitoring the difference between two consecutive values of compressive strength obtained and also monitors the difference between highest and lowest value of the strength obtained in a specified grade of concrete of different batches of the same slot produced from same mix design. The coefficient of variation presented in column (9) is a good indicator of the degree of quality control maintained by the plant. As per the results obtained the coefficient of variation varies from 0.06 (Case 2) to 0.164 (Case 1). The average coefficient of variation of Case 1 is 0.118, Case 2 is 0.068, Case 3 is 0.131 and of CASE 4 is 0.116.
These results reflect the fact that amongst the four cases under analysis best quality control is maintained by the plant of Case 2, followed by Case 4, Case 1 and Case 3.
Proposed Quality Control Model for Indian RMC Industry
Based on the analysis carried out in this present research, a Quality Control Model which can be widely accepted by the Indian RMC industry has been proposed. The schematic representation of this model is presented in Figrue. 1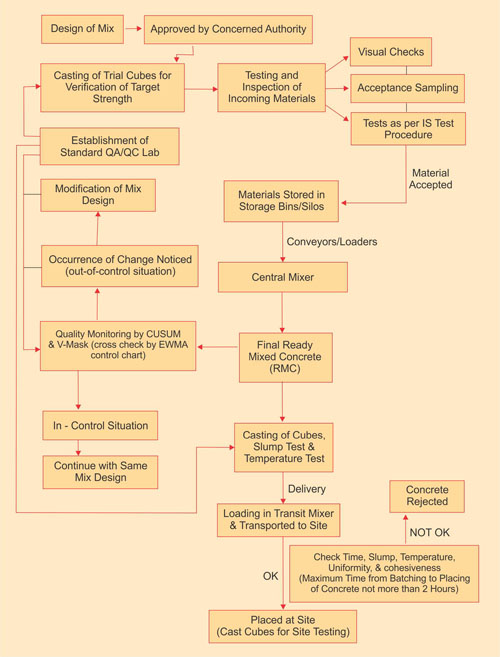
Quality Assurance (QA)/ Quality Control (QC) Team
The QA /QC team for the proposed model should be headed by a QA/QC Manager. He is the key person involved in the decision making process. He should be assisted by QA/QC Incharge whose job is to co-ordinate and implements the QA /QC guidelines and test procedures for the entire batching plant. Minimum two QA/QC Engineers should assist the incharge. The engineers should be involved in physically conducting the tests of the incomming raw materials and the final product ie. RMC. They should be physically present to check that all the equipment are calibrated properly. Also they should carry out the daily or weekly quality monitoring as per the proposed model. The QA/QC Incharge will take decisions about the assignable causes noticed during the monitoring phase. At least four lab technicians should be employed per plant to carry out the sampling and testing.Establishment of Standard QA / QC Lab
A standard QA /QC lab with latest testing equipment should be established at the plant.Testing of Raw Materials, Fresh Concrete and Hardened Concrete
The incoming materials particularly raw materials like cement, coarse aggregate 10mm, coarse aggregate 20mm, fine aggregate, admixtures should be subjected to testing as per adopted acceptance sampling plan. A proposed scheme for the basic tests to be conducted for raw materials, fresh concrete and hardened concrete along with their frequency of testing is given in Table 3.


Operation as per Proposed Model
The design of the mix should be carried out by competent authority and should be approved by authorities like Indian Institute of Technology (IIT), National Council of Cement and Building Materials (NCCBM), Council of Scientific and Industrial Research laboratories, Concrete Technology laboratories of National Institute of Technologies etc. The approved mix design for each grade of concrete should be tested in the plant as per plant conditions by casting trial cubes. Any major deviations of strength should be immediately communicated to the concerned authority and necessary rectifications should be carried out immediately.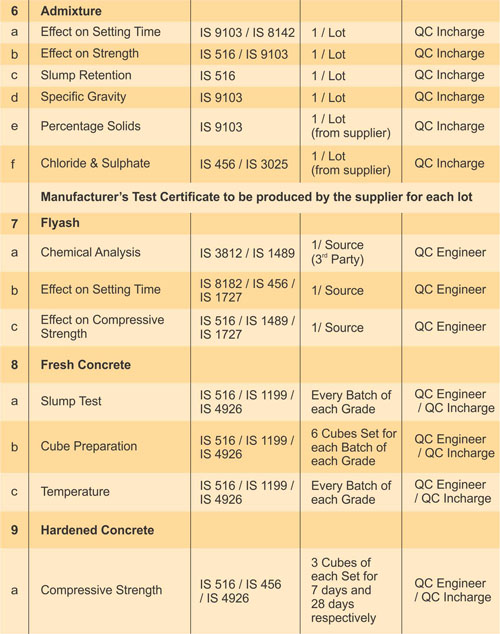
A standard QA /QC laboratory equipped with latest testing equipments should be established at site. Minimum two lab technicians should support two QA /QC engineers. The QA / QC engineers should report to QA / QC Incharge who in turn should report to QA/QC Manager. The incoming raw materials (cement, fine aggregates, coarse aggregates 10mm, coarse aggregates 20mm, flyash, admixtures etc.) should be tested with visual checks as per the testing procedures laid down by the respective QA/QC department of the plant. The raw materials found unsatisfactory in the visual checks should be rejected and as per requirement confirmatory tests should be conducted before rejection. The supplier should be immediately informed about the rejection of his material and should be warned so that if he repeats the same, his contract would be terminated. Standard tests for raw materials, fresh concrete and hardened concrete have to be performed as per Indian Standard (IS) testing procedures. The details of these tests along with the respective IS codes and frequency of testing is given in table
The materials qualifying in the respective tests are accepted and aggregates are stored in respective aggregate storage bins. Cement and flyash should be stored in cement silo and flyash silo respectively. It is desirable to transport the cement in bulkers and directly pump it into the silo through pneumatic pumps. The cement if required to be stored in godowns should be well protected from rains. The godown should be covered on all sides and should be provided with adequate size of lockable entry or exit gates. Cement should always be stored on a plain cement concrete base which is about 200 mm thick or brickwork base which is about 300 mm above the ground. The oldest lot should be used first. It is always better to cover the cement bags with polythene sheets particularly in pre-monsoon and monsoon seasons. The workforce involved in handling cement bags or bulk cement should compulsorily be provided with personal protective equipments like safety helmets, safety shoes hand gloves and safety goggles. During pumping of cement into the silos the workmen should take utmost care so that the cement due to back hammering effect does not create major accidents like severe eye injuries. The workmen should compulsorily wear safety goggles and helmet while working with the pneumatic pumps.
The stored raw materials should be weighed (as per the weights of design mix) in the respective weigh hoppers fitted with load cells. These weighed materials through screw conveyors or loaders should be transported to the central mixer. Measured quantity of water through water meter and admixtures through admixture dispensers should be added into the central mixer after dry mixing of cement, flyash, coarse aggregates and fine aggregates. After addition of water and admixtures the final mixing should be carried out for about 30 seconds. The entire loading process takes about 90 seconds. Thus the ideal cycle time for each of concrete is about 120 seconds. The capacity of each batch may be of 0.5 m3, 1 m3 and 2 m3 depending upon the capacity of the plant.
The RMC thus produced have to undergo tests of fresh concrete like slump tests and corresponding temperatures of the concrete should be noted. If the concrete is produced in summers when the outside temperature is about 40 to 45 degree Celsius the drum of the transit mixer should be covered with wet jute cloth and desirably some ice cubes should be added to the fresh concrete. The slump of the mixes to be placed by concrete pumps should be at least 180mm to 200 mm at the time of pumping. Thus use of flyash in the mix is always desirable for pumpable mixes as flyash due to ball bearing action increases the pumpability of a mix. The slump of concrete to be placed by crane and concrete bucket or by elevator hoists should be about 100 to 120mm at site. Minimum 6 cubes (150mm x 150mm x 150mm) should be prepared for each batch of concrete produced as per standard procedures. The cubes should always be casted in a cool shady place and in no case should the casting procedure be carried out under hot sun. The cubes should be air cooled for about 24 hours and then placed into the curing tank for curing. The curing tank should be completely filled with water and desirably should contain different compartments for 3 days, 7 days and 28 days testing. The fresh cubes should be marked with the specified grade of concrete, date of casting and should be placed in a jute gunny bag and after tying the mouth of the bag and putting a plastic tag of the grade and date of casting of the concrete, the bag should be placed in the desired compartment of the curing tank. Generally if the requirement is that the concrete needs to be tested for 7 days and 28 days compressive strength, then 3 cubes should be packed in the jute gunny bag and placed into the compartment for 7 days curing and other 3 cubes should be placed in the compartment for 28 days curing. As per client requirement if 3 days strength also needs to be tested then total 9 cubes should be casted. Following a systematic procedure of casting and curing the cubes improves the degree of quality control of the plant. The cubes should be tested in hydraulically operated compressive strength testing machine and desirably the manually lever operated strength testing equipment should be avoided. Care should be taken to ensure that the cube is uniformly seated before application of the load and the rate of loading should be uniform and desirably 140 kg /cm2/min. The failure load of the three cubes should be noted separately and the corresponding compressive strength should be calculated. The average of three cube strengths can be considered as the observed strength of a sample.
The fresh concrete thus prepared is loaded in transit mixers and transported to respective sites. The time from batching to placing of the concrete at the site should not exceed 2 hours. If the concrete is transported in summers the drum of the transit mixer should be covered with wet jute cloth and during extremely high temperatures ice cubes should added in the concrete. No additional water should be added in the concrete enroute. Before placing, the concrete should be tested for desirable slump, temperature, uniformity and cohesiveness and if found ok should be placed at the respective site.
The 7 days and 28 days strength obtained should be monitored using CUSUM and V-mask. This technique should be used as a daily monitoring tool and when the results of about four to five samples are obtained the data should be plotted using Excel / SPSS software where the x- axis of the graphical representation of the CUSUM plot (mean strength, range and correlation) represents sample number and the corresponding y- axis represents the CUSUM value in N / mm2 or Mpa. The V-mask plotted in a transparent paper should be superimposed on the obtained CUSUM plot by placing the lead point of the mask on each and every obtained CUSUM value starting from the first sample. If the plot remains within the boundary of the mask, the process is in control. If the plot crosses the boundary of the mask, a significant change has occurred in the process mean and an action is required. The root cause of the problem need to be investigated and accordingly action should be taken. A change in cement content can be calculated by the empirical relationship proposed by Dewar and Anderson (1988), and trial cubes can be casted and tested with the modified mix design value. The proposed Quality Control Model for Indian RMC Industry is presented in Figure 1.
Conclusion
RMC emerges to be an advantageous material in congested sites where setting up of a mixing plant is difficult. Presently construction industry including real estate developers are opting for RMC of grades M20, M25, M30, M35, M40, M50 and even higher grades. However, in absence of proper and effective quality monitoring systems in most of the batching plants in India, they are lagging behind from their western counterparts in terms of operational procedures, product quality and economy in manufacture of concrete. Hence there is a need to analyze the applicability of scientific quality monitoring techniques to RMC in India. The proposed Quality Control model for Indian RMC industry is a SQC based, simplified user friendly model, which if adopted, we think, would make Indian RMC industry much more organized and efficient in terms of production and operation.Acknowledgment
Author highly acknowledges the cooperation and help of the RMC batching plants of Ahmedabad and Delhi under study, in providing necessary information for this research work.References
- Dewar, J.D. and Anderson, R. (1988) Manual of Ready Mixed Concrete Blackie and Son Ltd., Glasgow and London.
- Box, G. (1994) “Role of Statistics in Quality and Productivity Improvement” Journal of the Royal Statistical Society, Series A, Part 2, pp. 209-229
- Keats, J.B. and Montgomery, D.C. [Editors] (1996) Statistical Applications in Process Control Marcel Dekker, Inc., New York.
- Montgomery, D.C. (1985), Introduction to Statistical Quality Control John Wiley & Sons, Inc, New York.
- Montgomery D.C. and Woodall, W.H. (1997) “A Discussion on Statistically Based Process Monitoring and Control.” Journal of Quality Technology Vol. 29, pp. 121-162.
- Sarkar, D. and Bhattacharjee, B. (2003) “Quality Monitoring of Ready Mixed Concrete Using Cusum System” Indian Concrete Journal, Vol 7, pp. 1060-1065.
- Woodall, W.H. (1986) “The design of CUSUM quality control charts.” Journal of Quality Technology, Vol. 18, pp. 99-102.
THANKS TO
Er.SP.ASWINPALANIAPPAN., M.E.,(Strut/.,)
Structural Engineer
Madras Terrace Architectural Works